Stand Back, Offspring does Science (Part 2)
by the Offspring Team
In the last two years, the Offspring has covered diversity-, discrimination- and inclusion-related topics in the academic world and adapted the mainly UK & US based phenomenon of the Awareness Months initiatives. Until last year we covered topics like mental health, LGBTQ+, ethnic diversity, disability and women in STEM, often collaborating with the Equal Opportunities group of the Max Planck PhDnet.
This year we decided for a similar, but different system. In the beginning of the year the Offspring team decided on topics that we felt are important and we would like to discuss further. Over the year we will have special editions about topics that we want to write about.
Hence, this April, Offspring brings you Science Communication Awareness Month. Since all members of the offspring team are scientists, we are proud to bring you what we do in Science. Our research aims broadly to deepen mankind’s understanding of the world. The only way we can do that is by efficient and effective communication. Science is not meant only for scientists, but for everyone to understand and trust facts and logic. Hence, this Science Awareness Month, gear up for some exciting science spanning varied topics heading your way, directly from the members of our team.
Check out Part 1 of the Stand Back, Offspring does Science here:
Sincerely,
The Offspring Team
Swati Subramanian
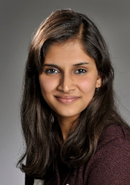
Ever wanted to learn a new skill such as juggling, playing the piano, or a new dance form and thought to yourself “I’m probably too old for that.”? The answer is, you aren’t! This is because our brain forms new connections even during adulthood. Of course, it takes longer to acquire new skills as one gets older. The phrase, ‘practice makes perfect’ is even more true as one grows older. There are plenty of people who show exquisite proficiency in a particular skill that they only began learning as adults. This is attributed, in part, to the process of myelination. To understand myelination, you need to imagine your brain as a large network of wires, called neurons.
These neurons are responsible for generating impulses called action potentials, and when you’re younger, they constantly break and form connections with neighbouring neurons, which gives rise to plasticity. Myelin is a fatty insulation around the axons of neurons, similar to the kind that your beloved phone charger has. In this way, myelin helps speed up action potential propagation. But there comes a point in one’s development when unbridled plasticity can be more harmful than beneficial. For example, uncontrolled plasticity can be detrimental for long-term storage of memories. Myelin is thus postulated to be a regulator of plasticity that creates order out of chaos. While myelination occurs at its peak during childhood and adolescence, it continues well into adulthood.
This is where my research lies. I’m trying to understand what kind of regulatory roles myelination and the cells that mediate it in the central nervous system, the oligodendrocytes, play in the developing brain with respect to memory retention, skill acquisition, and behaviour. Using well-established mouse models that either lack functional myelin in the entire brain or only in specific regions, I wish to study the effect of myelin on developmental plasticity. And if its absence indeed allows for an extended plasticity in the adult brain, what are its consequences on a molecular and on a cognitive scale?
Merel Wolf
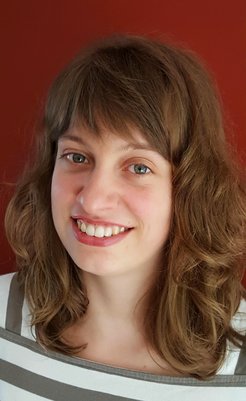
At the base of every form of communication lies an arbitrary connection between a signal -like a sound, a symbol, a gesture or movement- and the meaning that this signal represents. Many animals use specific signals to convey certain meanings. Meerkats, for example, have different calls for different types of danger: with specific calls, they can convey the type of predator (a sky-based predator or a land-based predator) and the urgency of the danger. Humans are exceptionally good at using signals to convey meaning: in their spoken languages alone, adults know the meaning of about 17 000 different words!
The connection between a signal -a word in this case- and a meaning, is obviously not something a child is born with. That means that all those 17 000 words and their meanings were memorized at some point in life! During their childhood, children show a steep growth in their vocabulary. But learning new words and their meanings does not stop once adulthood is reached: your vocabulary will keep growing through your entire lifetime.
Words can be learned in two distinct ways: by listening to someone talking, for example when having a conversation or listening to the radio, or by reading a text, be it books, newspapers articles or even food labels.
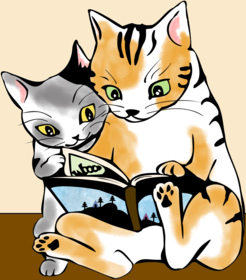
What’s interesting is that one of these learning paths -namely learning by listening to speech- is already present at birth, whereas for the other -learning while reading- the reading skill must first be acquired. Children learn to read fluently between the age of six to eight. This means that the ability to learn new words by reading is delayed by about six to eight years compared to the ability to learn by listening to speech.

In my research, I investigate whether learning new spoken words and meanings is more efficient than learning new written words and their meanings, or the other way around. If learning in one way is more efficient, why is that the case? Are there any inherent properties of the reading or listening process that make learning more efficient? Or is learning from spoken materials more efficient, because of the delayed experience with learning from written materials? Do you need to catch up to these six to eight years of experience? Or do adults show no difference in learning efficiency of spoken and written materials at all? Do you just need to become a proficient reader in order to learn new words efficiently while reading? If so, then at what age or what level of reading proficiency does learning new written and spoken words become equally efficient?
Raed Hmadi
Humans have two sets of each chromosome, inherited from father and mother, respectively. It is crucial that the same amount of output is generated from both chromosomes. Otherwise, abnormalities can arise, resulting in developmental failures and disease. Notably, sex chromosomes behave differently. In fact, females have two X chromosomes, whereas males have one X and one Y chromosome. In order to balance out the differences between the sexes, humans, and many other organisms such as fruit flies, developed an epigenetic mechanism commonly referred to as “Dosage Compensation”. During dosage compensation in humans, females randomly inactivate one of their X chromosomes (forming the Barr Body), in a process called “X Chromosome Inactivation”. In contrast, male fruit flies hyperactivate their single X (i.e double expression). A complex of proteins and RNAs, namely the MSL complex, carries out dosage compensation in fruit flies. Interestingly, different members of the MSL complex have been conserved throughout evolution from flies into mammals. However, their function in the mammalian system is poorly understood.
In my research, I am investigating the role of the MSL complex members in female mice. Even though the different members are conserved, their function varies between fruit flies and mammals. Instead of targeting an entire chromosome, the mammalian MSL complex might be involved in regulating gene dosage of individual genes. Using different experimental approaches, including CRISPR/Cas9 technology and next generation sequencing, we aim to understand and expand our knowledge on the mechanism of gene dosage regulation in mammals, through the lens of the MSL complex.
I never expected to be working on the Barr Body when I was still teaching undergraduate students during my MSc. in Beirut. Yet, after joining the Max Planck Institute in Freiburg, the beauty of dosage compensation and “X Chromosome Inactivation” caught my attention.
Vinodh Ilangovan
Time is important for us humans to carry out our social life. Is time important and relevant for living systems other than humans?
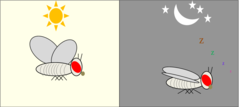
The continuous cyclic change between day and night influences life on Earth. Many forms of life, ranging from microscopic cyanobacteria to animals as large as blue whales, maintain an impressive internal time-keeping machinery called circadian (Latin: circa- approximately, diēm-day) clocks. Such living clocks or circadian clocks play an important role in preparing and matching the internal functions of our body to changes in the external environment. The hands of biological clocks can be easily understood by observing patterns of activity: rest, hunger and other physiological rhythms. I study biological rhythm in genes that dominate our daily lives in a rhythmic fashion. Such biochemical oscillators are present everywhere in our body, inside each cell generating the internal state of the circadian clock which can easily adapt to changes in external environment. Jet lag upon transcontinental travel is a manifestation that our internal clocks are not synchronised with the external environment.
The common fruit fly, Drosophila is an excellent model system to study the time-keeping machinery, as the genes share high degree of similarity with humans. Fruit flies exhibit robust activity: rest patterns with most activity during the day and a rest phase similar to sleep during the night. This non-invasive observation helps in understanding the internal state of the circadian clock
Constanze Depp
Why do our brains become vulnerable to neurodegenerative diseases when we grow old? A puzzling question that is yet to be solved. The most common neurodegenerative disease is the dementia Alzheimer’s Disease (AD) that affects almost 47 million people worldwide. In this disease, two protein species, namely Aß and tau, aggregate which is thought to be toxic to the brain and its neurons. Research in the field has mainly focused on how to clear the brain from these protein aggregates in order to cure the disease. But what if the protein aggregates are rather yet another symptom than the cause of the disease? We take an outsider’s perspective on Alzheimer’s disease and this view is shaped by the cell population that we study in the department of Neurogenetics and the MPI for Experimental Medicine: Oligodendrocytes.

These cells produce a membrane structure called myelin that wraps around neuronal processes to electrically insulate them - just like a electrical cable that also comes with an insulation. Myelin gives some brain structures their white colour which is why they are called ‘white matter’. Interestingly, white matter seems highly vulnerable to aging and defective white matter can be found very early in Alzheimer’s disease. In my PhD, I study this possible link of defective white matter and Alzheimer’s disease and I try to understand if white matter damage can be a trigger for the aggregation process. For this, I am using mouse models of Alzheimer’s disease in which I induce myelin damage by different means. For looking at the protein aggregates in the brain, I use a technique called light sheet microscopy in combination with tissue clearing (this makes the brain transparent!) which allows me to visualize the aggregates in the entire and intact mouse brain. With my PhD thesis, I hope to contribute to our understanding of brain aging and neurodegenerative diseases.
Aida Ahmadi
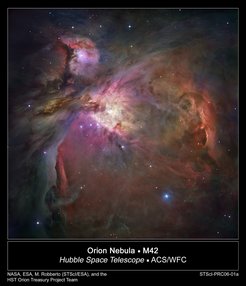
Have you ever wondered where we come from? While this can be a philosophical or religious question, I would like to tell you a short story about our origins and it all goes back to the stars. Stars are born from clouds of dust and gas that collapse under their own gravity. Once the centre of this collapsing material gets hot enough, nuclear fusion will convert hydrogen, which is the most abundant element in space, into helium and this provides the necessary energy for the star to shine.
During the formation of the Universe about 13 billion years ago, only light elements like hydrogen and a bit of helium were formed. So where does the carbon, oxygen, nitrogen, calcium, and many other elements that we find on Earth and in our bodies come from? When a star runs out of hydrogen, it starts to die as its fuel has been depleted. At this stage, a star like our Sun will start burning helium into carbon. Stars more massive than about 8 times our Sun are able to form even heavier elements like silicon and iron.
The most massive stars eventually die with an explosion called a supernova that expels these heavy elements into space. We are literally made up of the ashes of stars that died long ago! As important as these stars are to our very existence, our knowledge about their birth is lacking. For a long time, the existence of such massive stars was puzzling since it was thought that in the early formation phase when the star was just born and starting to radiate, the radiation from this newborn star would be so intense that it would push away any material that would still be falling in to feed it; so a star would not be able to accumulate enough mass to become much more massive than our Sun.
Yet stars that are more than 100 times as massive as our Sun exist. Theoretical and numerical works now support the theory that a high-mass star can form if a disk of material could be formed early on that could block part of the radiation from the star. Material could then fall from the cloud envelope onto this disk and then accrete onto the star through the disk. To confirm this observationally has been a difficult task because high-mass stars are rare, located very far, form in clusters, and live short lives making this accretion phase short-lived. With the advent of radio interferometers - arrays of telescopes observing together and achieving high resolutions - a handful of disks have been detected around infant high-mass stars.
For my PhD, I have made use of the Northern Extended Millimeter Array, which consists of eight 15-meter telescopes located in the French Alps, to observe 20 infant high-mass stars and study this stage in more detail and from a statistical point of view. We have found that indeed most of these stars seem to have disks around them. So the next time you wonder where the iron and oxygen that give your blood its red colour come from, you know exactly where! A very massive star that died violently expelling those heavy elements into space. And that star most likely formed with an accretion disk that provided it with fuel.